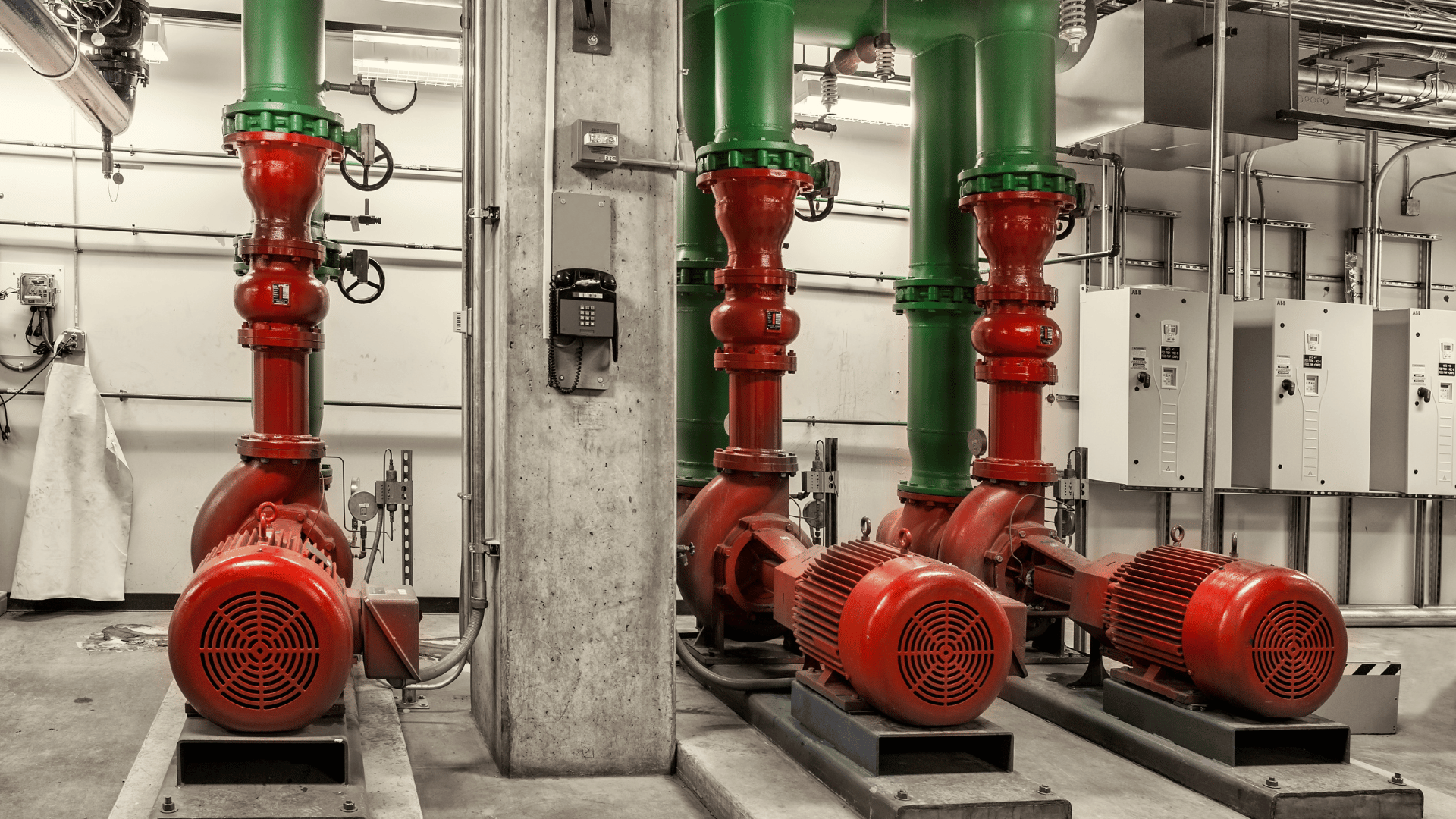
Electric motors are a primary consumer of industrial electricity. Using variable frequency drives (VFDs) to minimize motor energy consumption leads to significant energy savings. This article discusses how VFDs improve motor performance, ultimately reducing energy use and extending motor system lifespan. It also provides insights into selecting and implementing VFDs.
Author Contributor: E Tech Group Engineering Team Lead Ana Mircevska
Introduction
The US Department of Energy reports that machine-driven processes utilize 68% of the electricity in industrial manufacturing settings, with electric motors as the primary consumers. This statistic highlights the significant potential for electrical energy savings through technologies designed to improve motor energy efficiency.
To that end, variable frequency drives (VFDs) have become a critical technology addressing energy efficiency goals within manufacturing. VFDs allow for precise control of an AC motor’s speed and torque, optimizing the motor’s electricity use for the load – a particularly powerful technology for applications involving load variability. Regenerative VFDs further enhance efficiency by capturing and converting energy generated during deceleration back into usable power. Modular VFDs offer flexibility and scalability, allowing manufacturers to easily expand or upgrade their systems to meet changing operational demands while also contributing to both a smaller footprint and reduced electricity usage.
This article reviews the basic operation of a VFD, including regenerative and modular VFDs to provide an understanding for how these technologies provide remarkable motor control as well as energy savings while contributing to environmental sustainability goals. Some of the primary considerations in the selection process of a VFD for motor control are also discussed.
What is a VFD?
Recall that an AC induction motor’s speed is directly proportional to both the frequency of the incoming AC power supply and inversely proportional to the number of poles within its stator. Likewise, the torque generated by the motor is influenced by the applied voltage and the slip between the rotor and stator magnetic fields. Broadly speaking, a VFD provides a precise frequency and voltage to the motor to match the speed and torque requirements of the load. It does so by converting the incoming AC power supply to a different output voltage and frequency. By matching the motor’s speed and torque to the load requirements rather than running the motor at continuous full speed, a VFD saves electrical energy, therefore reducing operating costs.
A VFD is composed of three main stages: rectifier, DC bus, and inverter.
- Rectifier: The rectifier converts the incoming AC power source into DC power using diodes, or thyristors, for higher power applications. The output from the rectifier stage is a pulsating DC signal, meaning that the voltage/current never changes direction, but varies in magnitude.
- DC Bus: The DC bus smooths the incoming pulsating DC signal using capacitors and sometimes inductors. Capacitors store and release energy to eliminate voltage ripples, providing a steady and consistent DC signal. Inductors may be used to filter high-frequency noise. This stage ensures the DC power on the bus is stable for the final inverter stage of the VFD.
- Inverter: The inverter converts the smoothed DC signal from the DC bus back into an AC signal at a new frequency and voltage using pulse width modulation (PWM). PWM rapidly switches the DC signal on and off in a defined sequence using high-speed switching devices – typically insulated gate bipolar transistors (IGBTs), resulting in a simulated AC waveform at a new frequency. The duration of time that each pulse is held high (the duty cycle) affects the average voltage at the output terminals of the VFD that directly connect to the motor.
Soft Starting Capabilities of VFDs
By gradually increasing the output voltage to the motor, VFDs mitigate the issue of high inrush current that is typically seen at motor startup. This controlled voltage ramp-up results in a smoother, slower acceleration, which reduces mechanical stress on the motor, ultimately extending its lifespan.
Regenerative VFDs
While a traditional VFD can offer significant energy savings as described above, regenerative VFDs increase savings even further by capturing energy during deceleration that would otherwise be lost.
When a motor decelerates, the inertia of the rotor (due to its existing angular momentum) keeps it turning. Consistent with Faraday’s Law, this motion induces a current in the stator windings, causing the motor to function as a generator while slowing down, converting mechanical energy into electrical energy.
A traditional non-regenerative VFD engages braking resistors to dissipate the induced current, converting this energy into heat and therefore maintaining the voltage within operational levels on the DC bus of the VFD.
On the other hand, a regenerative VFD captures this induced current and returns it back to the DC supply bus of the VFD. From there, it can be used to power other parts of the system or converted back into AC power to be returned to the AC power grid. This feature is especially useful in applications where frequent braking occurs, resulting in significant energy savings.
The capability of returning energy to the power grid requires an active front end inverter stage. The active front end converter can convert both AC to DC power as described in a traditional VFD inverter stage, but also DC to AC power as required to transfer energy back onto the AC power grid.
Modular VFDs and Regenerative Braking
Modular VFD systems allow multiple motor control modules to share a singular active front end of a VFD. Each individual motor module is responsible for generating the appropriate average voltage and frequency to control its own motor’s speed and torque.
A modular VFD system allows for energy generated in a motor’s deceleration phase to be captured and returned to the common DC supply bus for a different motor module to use. This arrangement is especially helpful for systems that use multiple motors that decelerate and run during similar timeframes, allowing the decelerating motor to act as a generator for the active motor.
Modular regenerative VFDs also reduce energy costs by reusing the energy required to slow down a motor. The active front end of the modular VFD can be sized smaller, resulting in a reduction of physical dimensions and cost.
Example: Implementing VFDs to Control Payoff and Tension Reels for Energy Savings
An example application involving modular VFDs with regeneration capability includes a system with two motors: one operating a payoff reel and the other managing a tension reel. In industrial applications, a payoff reel dispenses material which is then processed and often rewound. A tension reel pulls the material forward to maintain tightness. The payoff reel must control the rate at which it dispenses material to avoid sagging or tearing during processing. As a result, it must often be decelerated to pull back on the material, placing the motor in regenerative mode. At the same time, the tension reel is often pulling forward. When a modular VFD system is implemented, the energy captured during the braking process of the payoff reel is transferred directly back to their common DC bus, allowing that energy to be used by the tension reel, optimizing the energy efficiency of the system.
AC Induction Motor Fundamentals
AC induction motors, also known as asynchronous motors, are the most widely used type of AC motor in industrial applications due to their reliability and efficiency. With fewer moving parts and a simpler design, induction motors are both more durable and typically require less maintenance than their DC motor counterparts. While both motor types have their specific appropriate applications, AC induction motors often provide a more cost-effective solution for continuous-duty work.
AC Motor Components
A basic AC motor consists of two main components: the stator and the rotor. The stator is the stationary part of the motor and contains coils of wire, or windings, that produce a rotating magnetic field when an alternating current (AC) is applied. The speed of this rotating magnetic field is determined by the frequency of the AC power supply and the number of poles in the stator windings, where a pole refers to each magnetic north and south pair created by the winding configuration. The rotor, located inside the stator, is the rotating component that turns in response to the stator’s magnetic field. It is made of conductive bars arranged in a squirrel cage shape.
AC Motor Basic Operation
The rotating magnetic field from the stator induces currents in the rotor bars (as described by Lenz’s Law), which in turn generates a magnetic field within the rotor that interacts with the stator’s magnetic field. Consistent with Faraday’s Law of electromagnetic induction, this interaction results in a torque on the rotor, causing it to rotate and therefore result in mechanical motion.
An AC induction motor’s rotor always rotates at a speed below that of the rotating magnetic field within the stator. The difference in rotational speed between the stator’s magnetic field and the rotor is called slip, which is responsible for the torque on the rotor.
Upon startup, slip is at a maximum, inducing sufficient rotor currents to generate a strong magnetic field necessary for high starting torque. As the rotor accelerates due to this torque, the slip begins to decrease. The motor reaches a steady-state where the slip stabilizes at a rate that is slower than the stator’s magnetic field, but sufficient to balance the mechanical load on the motor with the electromagnetic torque produced.
AC Motor Energy Usage
Torque and speed are both critical factors in determining the overall energy usage of a motor. Torque directly influences energy consumption. Higher torque demands require more current and therefore more electrical energy to overcome load resistance. Conversely, speed plays a pivotal role in a motor’s efficiency, typically peaking near its rated speed. Running a motor much below or above this speed can lead to inefficiencies due to disproportionate increases in energy consumption relative to mechanical output. Therefore, optimally balancing torque and speed, in alignment with operational demands and load conditions, maximizes the efficient conversion of electrical energy into mechanical work which defines motor efficiency.
VFDs in the Manufacturing Environment
By adjusting the frequency and voltage supplied to the motor, VFDs control a motor so that it operates at the specific speed and torque required for the current load. This makes VFDs particularly suited to optimizing energy use in variable load applications. Some examples include the following:
- Pumps, Fans, and Blowers: Power demand is proportional to the cube of the motor’s speed in a fluid-based system. Reducing the speed even a small amount results in significant energy savings. VFDs are well suited to control fluid flow rates, particularly in systems that do not always require the same speed of operation. As an example, in a HVAC system, a VFD can precisely control the amount of airflow required for maintaining a particular temperature setpoint.
- Conveyors/Material Handling: VFDs are used in conveyor applications to set the speed of the conveyor to precisely match the production rate, optimizing its energy consumption as well as the production rate of the system.
- Mixers/Agitators: By adjusting the speed to match the specific requirements for a process, VFDs optimize energy consumption and can also improve the overall consistency of the product by not overmixing.
Selecting a VFD
Selecting a VFD for a specific application involves matching it to the motor’s specifications. The motor nameplate provides this information, including:
- Motor Type: Verify that the type of VFD is compatible with the type of motor as different types may have different requirements. While several types of motors can be driven using a VFD, in general, “inverter duty” motors are specifically designed to do so.
- Horsepower: The VFD needs to handle the motor’s power requirement. Therefore, the VFD’s power rating should meet or exceed the motor’s horsepower rating.
- Service Factor: The motor’s service factor is an important consideration. According to NEMA MG1 and IEC 60034 standards, an AC motor with a service factor of 1.15 can sustain up to 115% of its rated horsepower under certain conditions. This means the VFD should be capable of handling this increased demand without overheating or tripping.
- Phase: The motor can be either single phase or three phase. The VFD must correspond to this specification. Note that three phase VFDs may use delta or wye configurations.
- Maximum Current, Torque, Speed: These parameters dictate the operational limits of the motor. The VFD must be able to supply sufficient current at varying speeds and manage the torque required. This is especially important during startup because it is often the state that requires the highest current and torque to overcome the combined inertia of the stationary motor and attached load.
Additionally, consider the following:
Current Overload Capability: The overload capacity of a Variable Frequency Drive (VFD) is an important specification that indicates how much additional current the drive can provide beyond its rated capacity for a limited duration.
Common overload ratings include:
- 150% for 60 seconds: Many VFDs can handle this level of overload for short durations.
- 200% for 3-10 seconds: Some drives can support higher overloads for very short periods, suitable for applications requiring high starting torque.
Drive Control Communication Protocol: Many VFDs come with a built-in display screen for direct control. However, if the VFD will be controlled by a PLC, verify that both the VFD and PLC support compatible communication protocols (e.g., Modbus, Profibus, Ethernet/IP).
Braking Capability: Because of the excess electrical energy that is generated during a motor’s deceleration, the braking capability of a VFD must be evaluated to ensure the drive can effectively manage or dissipate this energy. Consider the following:
- Deceleration Requirement: Evaluate the timeframe in which the motor needs to stop.
- Generated Energy During Braking: During deceleration, the kinetic energy of the motor must be dissipated. Since the motor is a rotating body, its kinetic energy can be found using the following formula:
- E= 1/2lw2 ; where E is energy measured in Joules, I is the moment of inertia (kg*m2) of the rotor and load, and w is the angular velocity in radians/s.
- P= E/t ; where P is power measured in watts, E is energy in Joules, and t is time in seconds.
- Braking Power: The VFD’s specifications often indicate its braking capability in terms of power. Should the power generated during the application’s braking event exceed the braking capability of the VFD, dynamic braking resistors will need to be added to dissipate the excess energy safely based on the braking power calculated above.
- Braking Duty Cycle: Evaluate the frequency of braking events to ensure that the system and/or braking resistor can handle the thermal stress generated by the rate of deceleration events of the motor.
Regenerative VFDs and Total Harmonic Distortion
Total harmonic distortion (THD) is a measurement that quantifies the distortion of a waveform in comparison to its ideal sinusoidal form. THD is expressed as a percentage and indicates the amount that the waveform deviates due to harmonic frequencies beyond the fundamental.
When integrating regenerative VFDs with the power grid, compliance with the IEEE 519 standard for permissible levels of total harmonic distortion must be met. This standard specifies the acceptable limits for harmonic currents and voltages introduced by electrical equipment, including regenerative VFDs. To meet these standards, additional filtering devices may need to be installed on the incoming side between the power supply and the VFD to maintain compliance with regulatory requirements.
Cable Selection
The fast switching that occurs with pulse width modulation (PWM) results in signals with high frequency content. When the length of the cable approaches a significant fraction of the wavelength of the highest frequency component of the signal (which is often the case with the high frequencies generated by PWM), the cable’s transmission line characteristics must be considered. Some cable considerations for high frequency operations include:
- Shielding: Use shielded cables to reduce electromagnetic interference and maintain signal quality.
- Grounding: Ensure proper grounding of cable shields to prevent electrical noise and safety hazards.
- Cable Length: A suitable cable has a maximum operating length that minimizes transmission line effects including voltage reflections, resonance, and voltage drops.
- Installation Practices: Install cables avoiding sharp bends, stretching, or compression that could damage the cable and affect its performance.
Inverter Duty Motor
For best compatibility with a VFD, a motor must be inverter duty rated, rather than general purpose. Inverter duty motors are designed with better insulation so they can withstand the voltage spikes and fast switching frequencies generated by the PWM of the VFD.
Addressing Parasitic Current Generation
PWM in VFDs can generate parasitic currents, often referred to as bearing or common mode currents, which can flow through the motor’s bearings. These currents can cause damage to the bearings over time and can also interfere with other nearby components such as encoders. To address this issue, it is recommended to select a motor that includes a shaft grounding ring. This ring diverts the common mode current away from the bearings and into a grounded path, protecting both the motor and connected equipment.
Concluding Remarks
The integration of VFDs into manufacturing not only improves motor control but significantly boosts energy efficiency. Generating precise management of motor speed and torque, VFDs control the motor to match process needs and minimize unnecessary energy consumption. Regenerative and modular VFD technologies further increase energy efficiency benefits by recovering and reusing the energy that would otherwise be lost to motor deceleration. As a result, VFDs reduce operational costs by reducing the overall energy required for manufacturing processes. As industries continue to emphasize energy efficiency and sustainability, the role of VFDs in achieving these goals becomes increasingly crucial, making them key components in the future of industrial automation and energy management.
Contact Us For Column
Reach out to us here and someone will respond within 24 hours.
"*" indicates required fields